Looking Through Nature’s Lens
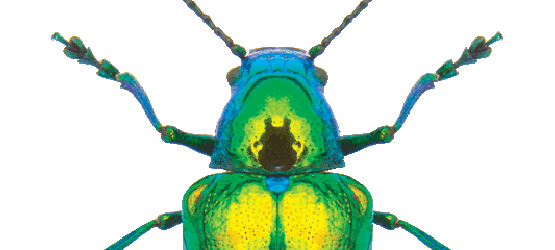
What do a beetle shell, a rat’s tail, and a microscopic worm all have in common? They each boast unique optical properties that are very much in demand for modern-day technology—with practical uses in everything from sunglasses to computer screens to lasers. Those optical properties usually require a lot of money and energy to produce, often with highly toxic metals and chemicals. Yet Mother Nature creates such effects for free, with little energy and remarkably simple structures and life forms. Scientists have yet to understand fully how she does it, but physics professor Jenny Magnes aims to find out.
Magnes specializes in applied optics, the study of optics for practical
—often technological—uses. When she began teaching at Vassar, she looked for applied science projects she could share with her students.
One thing is clear, says Magnes: “Nature has some impressive stuff that we want to replicate.” Its optics have been shown to change the polarization of light (as many sunglasses do), manipulate light wavelengths (useful for applications in computer monitors and lasers), and have become an important component in two-photon microscopy (an imaging technique valuable in the medical field that uses collagen). The non-linear optical properties of collagen were first discovered in the rat tails.
Magnes is interested in natural optics that display some level of quantum behavior. Consider common beetles. “They have very pretty colors, all kinds of colors,” she says. “But really they have no pigment in their shells.” The colors we see in iridescent beetle shells are the result of inherent optical properties, such as thin film interference. Frequency doubling can also occur in beetle shells, it takes longer wavelengths of light and other forms of electromagnetic radiation and produces shorter wavelengths. The process is used in the production of certain lasers. Manufactured crystals used in that process cost thousands of dollars, but a beetle’s shell doubles frequencies naturally.

In addition to being expensive, crystals require high inputs of energy. Crystals that turn longer-wavelength red light into shorter-wavelength blue light, for example, typically need a lot of power. “Beetles do it with very little power. They’re very efficient. But we don’t know how that works,” says Magnes.
We don’t know now, but maybe someday we will. Products produced with natural optics would certainly make disposal a greener operation. “With an organic display, or an organic computer, you can then throw it on your compost instead of having to worry about it as hazardous waste,” says Magnes.
Magnes takes a closer look at technology—both natural and human-made—in her Vassar Applied Optics Lab, where she works with students. One especially popular course is Lasers, Technology & Teleportation. It takes her applied optics and other applied sciences and brings them to bear on all sorts of real-world and science fiction technologies: GPS (How does it work?), energy consumption (How do you calculate your electric bill, and how do you know if the utility company cheated you?), lasers (How much laser do you need to blow something up, and could the Death Star in Star Wars actually be built?), mind reading (Is it possible?), and nuclear radiation (What was the real threat at the Japanese reactors in the wake of the 2011 earthquake and tsunami?).
“Their experience working on these real-world projects is invaluable,” says Magnes. “Once they understand how to apply the science to a particular topic, they can translate that elsewhere.”
One of Magnes’s latest efforts is a National Science Foundation-funded grant project with collaborator Kate Susman, a biology professor at Vassar. They’re looking at C. elegans, a nematode (roundworm) that lives in the soil and which grows up to about 1mm long. “They only have about 300 neurons, but can do a lot of things—learn, sense light, tell direction, know gravity,” explains Magnes. “They’re very interesting to the neuroscience community, yet we know very little about how this microscopic life moves.”

Magnes is using diffraction of light to figure that out. It’s difficult to observe C. elegans under a traditional microscope. “You can watch it under the microscope in a flat focal plane, but that’s not how it moves in real life,” she explains. She is planning to use quantum optics (ghost imaging) to observe the worms in a soil like environment.
Magnes’s novel idea is to use diffraction—a pattern of light and dark spots created by laser light—to look at what C. elegans is doing. She compares still images of C. elegans to the diffraction pattern the worm creates when it moves. If she assembles a robust enough library of those paired images, she can essentially create an animated flipbook that will give researchers a clearer window into how these tiny organisms move. Her research will better enable biologists such as Susman to learn about C. elegans for the sake of neuroscience.
Such innovative work has caught the eye of the Journal of Visualized Experiments, the first peer-reviewed video journal, which came to campus this summer to film Magnes performing her experiments. The journal is like a YouTube channel for scientists—instead of trying to decipher dense instructions in scientific literature, viewers can watch scientists as they work. Now who’s under the microscope?